SUBLIME: Single-use battery-less wireless temperature sensor for lyophilization process
キーワード:Lyophilization, Freeze-drying, sublimation, Real time, Wireless probe, single-use, PAT tool, GMP, battery-less, wireless power
凍結乾燥プロセスにおける1次乾燥中の昇華面(未乾燥部と乾燥部の境界)での状態は重要なパラメーターであり,凍結乾燥レシピの開発,検証,および生産の堅牢性維持に与える影響は相当なものである。特に参考文献(1)に概説する新しい医薬品の製造管理および品質管理の基準(GMP)への対応のため,凍結乾燥プロセスが一層複雑になっている。本論文では,SUBLIMEという名称の新たなプロセス分析技術(PAT)ツールを取り上げた。本ツールは,バッテリー不要,使い捨て可のワイヤレスプローブを採用し,昇華面の温度をリアルタイムでモニタする。この革新的なツールは,凍結乾燥炉への自動搬入搬出システムを用いることで,これまで困難だった温度モニタの利用を簡略化しただけでなく,相互汚染リスクも軽減する。
Introduction
The pharmaceutical industry demands sophisticated technical solutions that align with stringent quality controls to ensure the safety, efficacy, and production of pharmaceutical products. The recent updates in GMP conditions, specifically outlined in Annex 1, emphasize the need to reduce or prevent manual interventions in the manufacturing processes. This requirement encourages the demand for automatic loading and unloading systems, as they play a pivotal role in minimizing the risk of cross-contamination.
Moreover, maintaining specific conditions for the product throughout the lyophilization process incurs substantial production costs. In this context, a PAT tool for continuous monitoring of critical parameters during lyophilization becomes imperative. This tool facilitates precise monitoring of the process, minimizing the risk of product damage or loss. The objective is to develop a solution that aligns with the latest GMP conditions, addresses the challenges posed by automated systems, and enhances the overall efficiency and reliability of the lyophilization process.
Background
Lyophilization, commonly known as freeze-drying, is a high-value drying process integral to the preservation of pharmaceutical and biological products. The process comprises three main phases: freezing, primary drying, and secondary drying. The primary drying phase involves placing the product under vacuum conditions, causing the solvent to sublimate from a frozen solid state to a vapor state. This phase, particularly the advance of the sublimation front, is crucial for achieving proper drying and preserving the quality of the product.
The sublimation front is the region where phase change occurs, and maintaining the temperature at this interface below the critical temperature of the product is paramount. Failure to do so could lead to product damage and the loss of critical quality attributes (CQAs).
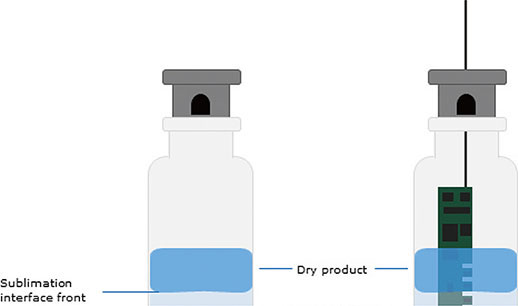
Figure 1.Sublimation interface front during primary drying
The product temperature during lyophilization is influenced by various factors, including the freeze-drying recipe, shelf temperature, chamber pressure, and other process parameters. While existing solutions, such as wired thermocouples or wireless temperature probes, can monitor product temperature, they often fall short in monitoring the sublimation front temperature due to the downward movement during the process.
Wired or battery-operated wireless probes are challenging to manage in systems equipped with automatic loading and unloading. Monitoring the sublimation front interface during production batches, especially during scale-up or optimization activities, becomes a complex operation when automatic systems are in place. The prevalence of such systems is increasing rapidly, with around 80% to 90% of new equipment for sterile applications incorporating automatic loading and unloading features. This necessitates addressing compatibility with GMP requirements, operating in strict aseptic conditions, and minimizing the risks of contamination and cross-contamination.
The challenges posed by automatic loading and unloading systems extend beyond GMP requirements. These systems must be robust, ensuring seamless operation and production continuity. The ability to address issues promptly and save production in case of failure is crucial. Introducing and retrieving wireless temperature probes into the freeze dryer feeding line automatically is another challenge, particularly when human intervention is minimized. Existing devices placed between the filling line and freeze dryers can insert sensors with minimal disruption to the loading process and retrieve them during unloading operations.
However, in the face of new GMP requirements, limited information during the lyophilization process, potential risks between products and operators, and the dedicated time required to manipulate existing temperature probes, there is a pressing need for a new solution. The traditional approach of adding product temperature sensors manually once the freeze dryer is fully loaded is no longer viable. Thus, a new solution is imperative to address these challenges and enhance the efficiency and reliability of the lyophilization process.
Challenge resolution
In response to the evolving GMP requirements, the lack of information during the lyophilization process, potential risks between products and operators, and the extra time required for manipulating existing temperature probes, a new solution has been developed. This solution involves the introduction of a single-use battery-less wireless temperature probe capable of monitoring the advance of the sublimation front interface in real-time, ensuring accurate temperature tracking during the freeze-drying cycle.
This novel probe is designed as a single-use, disposable device with no built-in battery or internal power supply. The energy required for operation is harvested from an antenna placed inside the lyophilization chamber, connected to a Gateway platform positioned outside. This antenna serves a dual purpose, functioning as both the power generation antenna and the communication antenna. This design choice reduces the number of elements that need placement inside the chamber, streamlining the overall configuration.
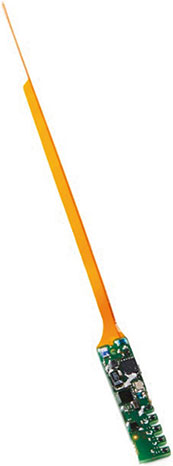
Figure 2.Sublimation interface front temperature single-use wireless probe
When remotely powered from the gateway, the wireless probe measures the temperature using five different measurement points. These points are strategically positioned along the device in a single line with a pitch of 2.5mm, enabling the real-time monitoring of the sublimation front temperature during the drying phase. The absence of cables or batteries is made possible through a patented power transmission technology. The main body of the probe consists of a printed circuit board covered with an FDA-compliant coating.
The probe’s electronic circuit incorporates a capacitor that collects the necessary power from the generator unit (in the gateway) through a carrier wave sent to the antenna inside the chamber. This design eliminates the need for an external battery, avoiding potential temperature variations caused by heat emission. Furthermore, it reduces mechanical interferences during loading and unloading processes, as there is no requirement for extra space to accommodate a battery.
Once sufficient power is harvested, the probe can read the product temperatures along the drying cake at the five measurement points. The information is then transmitted wirelessly back to the gateway via the integrated 2.4 GHz antenna. Notably, no pairing or connection between the probe and the gateway is required for data exchange. The configuration of power transmission and communication can be customized, allowing adjustments to the periodicity of measurements based on the lyophilization cycle’s duration.
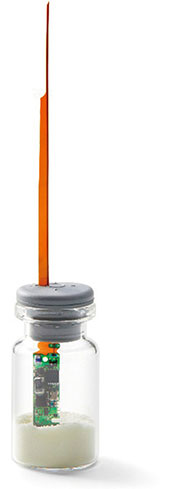
Figure 3.Correctly dried product after lyophilization cycle
The gateway features an internal CPU with a timer circuit that manages the measurement time interval, controlling both power emission and temperature reading duration. Beyond transmitting temperature measurements, various electrical statistics from the probe’s electronic circuit module, such as voltage value obtained by the power generation unit and reception intensity at the wireless unit, are wirelessly sent back to the gateway. Additionally, the sensor interface circuit may transmit abnormal states, such as short circuits or disconnections, as additional data.
All the received data can be displayed at the freezedryer Supervisory Control and Data Acquisition (SCADA) system via an OPC UA protocol. The SCADA interface provides a comprehensive overview of the freeze-drying process by merging temperature measurements from the probes with data from the freeze dryer, including shelf control temperature, shelves inlet and outlet temperatures, vacuum in the chamber, condenser temperatures, and more.
Comparison with thermocouples and endpoint determination
In order to showcase the performance of Sublime probes and highlight their significant advantages over existing solutions for monitoring product temperature during lyophilization, a comparative analysis was conducted between Sublime sensors and thermocouples (both internal and external) positioned at the base of the product. Figure 4 illustrates the cycle trends of a sucrose lyophilization process, featuring an internal thermocouple (depicted in blue), an external thermocouple (depicted in grey), and the bottom measurement point of a Sublime sensor (also depicted in blue). All three sensors are strategically positioned in contiguous central vials.
Upon examination, notable differences in the data from the three sensors become apparent. The thermocouples consistently exhibit higher temperatures overall. Both internal and external thermocouples appear to measure the bottom temperature and detect the sublimation front less effectively than the Sublime sensors. This discrepancy may be attributed to the inherent difficulty in precisely positioning thermocouples at the exact center of the vial, resulting in their measurements incorporating a larger portion of the dry layer, which inherently possesses a higher temperature.
Despite the fact that all three sensors roughly indicate a similar sublimation endpoint by stabilizing at comparable times, the behavior of the product at the bottom of the vial appears to be more accurately represented by the Sublime sensor. The Sublime sensor's performance showcases a clear advantage in capturing the subtleties of the lyophilization process, particularly in comparison to thermocouples, thereby emphasizing its efficacy and precision in monitoring the critical phases of the freeze-drying cycle.
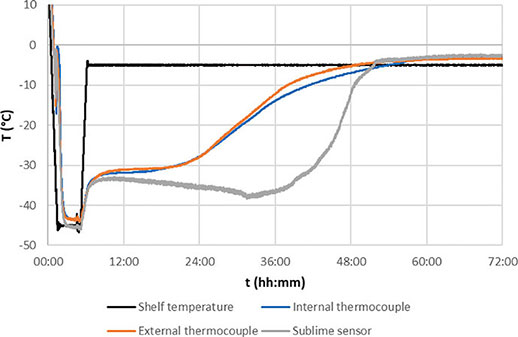
Figure 4.Sucrose 5% with 20R vials cycle trends with contiguous central sensors: int. thermocouple( orange), ext. thermocouple( blue), Sublime bottom measuring point( grey), shelf temperature( black)
Similar to thermocouples, Sublime sensors possess the capability to identify the conclusion of sublimation of the vial in which they are disposed. The stabilization of the bottom Sublime sensor temperature, nearing the shelf temperature, signifies the completion of sublimation in that specific vial. Another commonly employed method involves assessing the difference between a Pirani pressure gauge and a capacitance pressure gauge. When the reduction and stabilization of this gauge difference occur, it indicates the completion of sublimation (Tang & Pikal, 2004)
In Figure 5, the trends of a mannitol freeze-drying cycle are depicted, featuring pressure gauges, thermocouples, shelf temperature, and Sublime sensors. The thermocouple situated near the edge of the shelf (depicted in grey) stabilizes first, albeit by a small margin. A Sublime sensor positioned at the center of the shelf (depicted in blue) and a neighboring thermocouple (depicted in orange) both determine the endpoint at approximately the same time, slightly preceding the endpoint determination based on Pirani and capacitance gauges.
This aligns with the expected behavior, as the Pirani gauge signals the end of sublimation for the entire batch, whereas thermocouples or the Sublime sensor focus on the vial in which they are located. Vials positioned at the center of the shelf experience a slower sublimation process. Therefore, when determining the endpoint with temperature probes, it is recommended that the reference probe be centrally located, as demonstrated in this test. This strategic placement ensures a more accurate representation of the sublimation endpoint, particularly in scenarios where variations in sublimation rates exist across the batch.
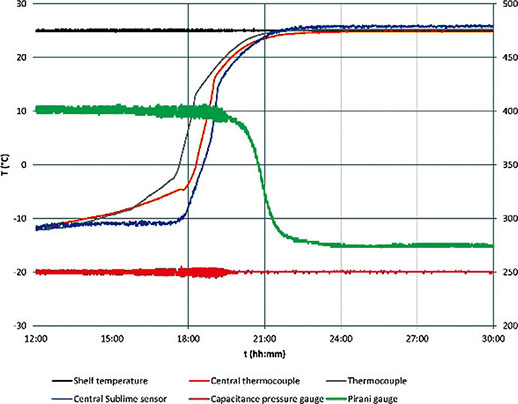
Figure 5.Mannitol 5% with 6R vials cycle trends: central thermocouple (orange), thermocouple (grey), Sublime bottom measuring point (blue), shelf temperature (black), Pirani (green), capacitance pressure sensor (red)
Batch homogeneity
The system also provides a visual representation of the disposition of temperature probes on the freeze dryer chamber shelves, positioned at various locations across the shelf stack. This feature enhances the representativeness and relevance of monitoring the sublimation front interface temperature. By seamlessly integrating temperature measurements from the wireless probes with freeze-dryer recipe variables, the sublimation process can be optimized, particularly during the critical primary drying phase. This optimization ensures that the product undergoes proper drying without surpassing its critical temperature, potentially leading to a reduction in overall drying time.
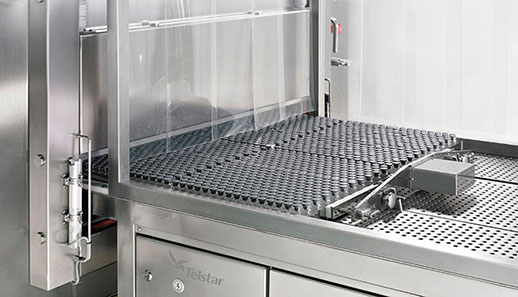
Figure 6.Vial loading shelfs
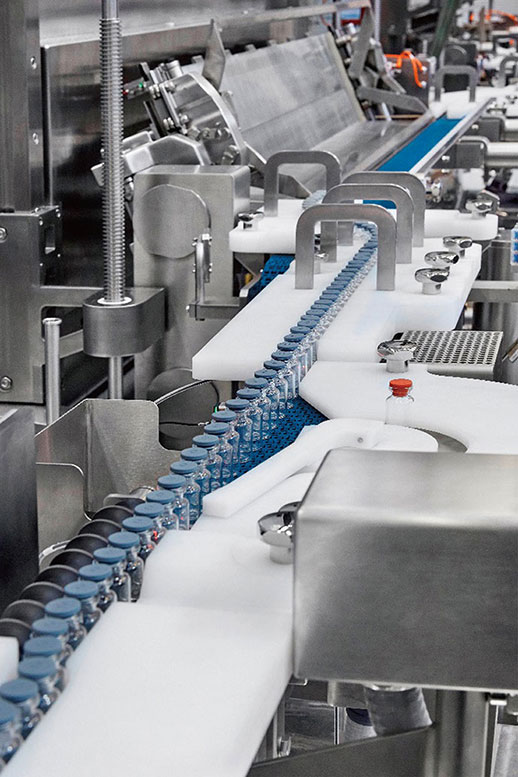
Figure 7.Vial automatic loading system
In a batch process, where homogeneity is a crucial consideration, it is well acknowledged that differences may exist between vials within the same batch. Various factors, such as the edge vial effect or the stochastic nature of spontaneous nucleation in the freezing phase, contribute to these variations. Vials placed at the edges, especially those near the door, receive more radiant heat, accelerating their sublimation process compared to vials positioned in the center.
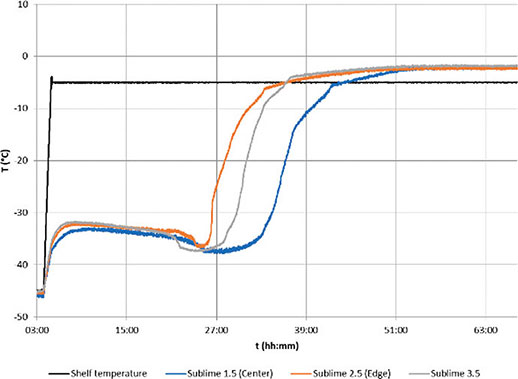
Figure 8.Sucrose 5% with 6R vials cycle trends with shelf temperature( black) and bottom measuring points of Sublime sensors: sensor 1( central, blue), sensor 2( edge, orange), sensor 3( edge, grey)
Figure 8 illustrates the measurements obtained from multiple Sublime sensors in a freeze-drying cycle performed with a 5% sucrose solution. The shelf temperature, depicted in black, is set at -5°C during the primary drying phase. In this specific test, the central vial is monitored with probe 1, represented in blue. The remaining Sublime probes in the graph are positioned closer to the edge of the shelf, with sensor 2 being the nearest to the edge. As anticipated, the edge vials exhibit the fastest sublimation rate, with a notable difference of up to 10 hours between probe 2 at the edge and probe 1 at the center of the batch.
Sublime sensors play a crucial role in enabling the observation and monitoring of process differences within the same batch. This capability provides valuable insights into the dynamics of sublimation across various vials, allowing for a more nuanced understanding of the freezedrying process and facilitating informed decision-making in optimizing product quality and drying efficiency.
Sublimation estimation rate
The sublimation process within a vial occurs primarily from the top to the bottom, with a lesser extent from the sides towards the center. Given the vertical movement of the sublimation front, the Sublime sensor offers a more direct observation of this phenomenon. Throughout the cycle, the sublimation front passes through each of the sensor’s 5 measurement points, moving from the top to the bottom of the product (Pikalx, Roy, & Shah, 1984). This dynamic is illustrated in Figure 9, depicting the freeze-drying trends of a 5% mannitol cycle alongside data obtained with a Sublime sensor.
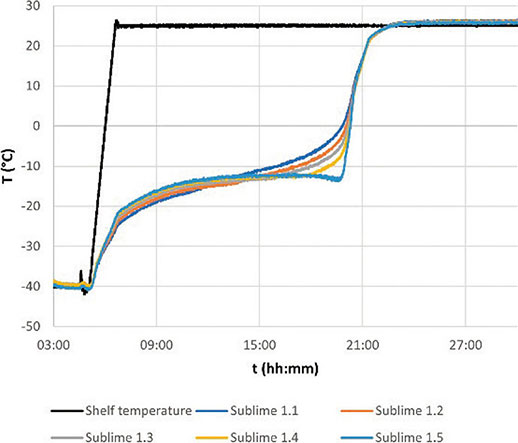
Figure 9.Mannitol 5% with 20R vials cycle trends with shelf temperature( black) and a central Sublime sensor: 1.1( top point, deep blue), 1.2( orange), 1.3( middle point, grey), 1.4( yellow), 1.5( bottom point, light blue)
In Figure 9, the Sublime data distinctly indicates the moment when the sublimation front traverses each measurement point. Each point becomes the coldest among the five, and a significant increase in the slope of the temperature function is observed as the front passes through that specific measuring point. As anticipated, the sublimation front is initially detected in the top-most sensor and sequentially passes through each point until reaching the bottom one.
This novel configuration allows for the calculation of the sublimation rate as the process unfolds, using the time difference between the detection of the sublimation front in different points of the sensor (Jiang, Zhu, Kodama, 2018). The calculation for an aqueous solution is as follows:
Where \(A_p\) is the cross-sectional area of the product using the inner diameter of the vials, \(Δ_h\) is the distance between the measuring points, \(C_{water}\) is the concentration of water in the solution, \(ρ_{ice}\) the density of ice and \(Δt\) the time difference between the detection of the sublimation front in different measuring points.
It’s important to note that this calculation excludes sublimation from the edge of the vial to the center and the release of bound water, which occurs during the secondary drying phase. With the data from the cycle represented in Figure 6, the sublimation rate from the second point to the third (middle) is calculated as 0.70 g/h, while from the fourth point to the fifth( bottom), it decreases slightly to 0.68 g/h.
Conclusions
The multi-point configuration of Sublime sensors introduces a new dimension to process understanding, tracking the sublimation front throughout the cycle and enabling sublimation rate calculation during primary drying. Have demonstrated remarkable accuracy in monitoring product temperature during lyophilization processes. Their versatility spans applications from laboratory-scale cycle development to real-time monitoring in industrial production. Compared to other existing technologies, Sublime consistently provide comparable temperature readings, particularly excelling in capturing the behavior of the vial bottom, enhancing freeze-drying process understanding.
Moreover, this solution determines the sublimation endpoint from the center vial, aligning with results from Pirani and capacitance gauges. This highlights their ability to observe differences between vials in the same batch.
With a single-use, disposable design, reduce manipulation time and risks, eliminating the need for manual recovery, washing, and sterilization. The innovative design with five independent temperature measurement points enhances real-time monitoring of the sublimation front interface, ensuring correct drying parameters and preserving product quality, representing a significant advancement in pharmaceutical production, offering automatic introduction and retrieval with wireless communication technology.
In conclusion, the integration of evolving technology, as exemplified by Sublime sensors, signifies a significant leap forward in ensuring the integrity, efficiency, and reliability of pharmaceutical manufacturing processes.
References
[1]European Comission. (2022) The Rules Governing Medicinal Products in the European Union Volume 4 EU Guidelines for Good Manufacturing Practice for Medicinal Products for Human and Veterinary Use. (https://health.ec.europa.eu/system/files/2022-08/20220825_gmp-an1_en_0.pdf)
[2]Foerst, P., Melo de Carvalho, T., et al. (2019). Estimation of mass transfer rate and primary drying times during freeze-drying of frozen maltodextrin solutions based on x-ray μ-computed tomography measurements of pore size distributions
[3]Jiang, X., Zhu, T., Kodama, T., et al. (2018) Multi-point wireless temperature sensing system for monitoring pharmaceutical lyophilization
[4]Kuu, W., Hardwick, L., Akers, M. (2006). Rapid determination of dry layer mass transfer resistance for various pharmaceutical formulations during primary drying using product temperature profiles.
[5]Nail, S., Jiang, S., Chongprasert, S., et al. (2002). Fundamentals of freeze-drying.
[6]Patel, S., & Pikal, M. (2013). Lyophilization process design.
[7]Nail, S., Jiang, S., Chongprasert, S., & Knopp, S. (2002). Fundamentals of freeze-drying. Pharmaceutical biotechnology, 14, 281-360.
[8]Patel, S., & Pikal, M. (2013). Lyophilization process design space. Journal of Pharmaceutical Sciences, 102(11), 3883-3887.
[9]Pikal, M., Roy, M., & Shah, S. (1984). Mass and Heat Transfer in Vial Freeze-Drying of Pharmaceuticals: Role of the Vial.
[10]Rambhatla, S., & Pikal, M. (2003). Heat and Mass Transfer Scale-up Issues During Freeze-Drying, I: Atypical Radiation and the Edge Vial Effect.
[11]Santafé, M. (2021). CASE STUDY Non-invasive product temperature monitoring as a recipe design and scaling up tool. (https://www.telstar.com/es/non-invasive-product-temperature-monitoring-as-a-recipe-design-and-scaling-up-tool/)
[12]Tang, X., & Pikal, M. (2004). Design of Freeze-Drying Processes for Pharmaceuticals: Practical Advice.
[13]Teagarden, D., & Baker, D. (2002). Practical aspects of lyophilization using non-aqueous co-solvent systems.
[14]Varshney, D., & Singh, M. (2015). History of Lyophilization. Springer New York.
[15]Ward, K., & Matejtschuk, P. (2019). Lyophilization of Pharmaceuticals and Biologicals New Technologies and Approaches.
[16]Wolkers, W. (2021). Cryopreservation and Freeze-Drying Protocols.
Author affiliation
Joel Nuñez Sanz
Azbil Telstar, S.L.U.
この記事は、技術報告書「azbil Technical Review」の2024年04月に掲載されたものです。
- 2024
- 巻頭言:期待
- 特集に寄せて
- DR・VPPのためのリソース割当計画の自動作成
- 新技術を「試し」「議論し」「育てる」ための新実験棟
- 予兆検知・診断技術の開発
- オンライン異常予兆検知システムの大規模プラント向け拡張機能の開発
- 生成AIのアプリケーションへの導入に向けて
- オフィスのABW実装による効果検証法
- SUBLIME: Single-use battery-less wireless temperature sensor for lyophilization process
- 赤外線アレイセンサシステムの開発
- 生産性向上の実現に向けたIO-Link™対応誘導形近接センサ用ICの開発
- savic-net™G5コンパクトモデル
- 空間の価値を高めるsavic-net™ G5 入退室管理システム
- 小型デジタル指示調節計
- 2023
- 2022
- 2021
- 2020
- 2019
- 2018
- 2017
- 2016
- 2015
- 2014
- 2013
- 2012
- 2011
- 2009
- 2008
- 2007
- 2006